The mould determines the strand crosssection. The liquid steel begins to solidify once it enters the mould to produce the strand shell, the thickness of which after it leaves the mould generally ranges between 10 and 30mm depending on the casting speed.
The mould is the only mechanical part that is exposed directly to the molten steel. It is probably the most important part of the machine and has to operate under severe conditions. It needs to create a homogeneous shell by efficient heat transfer. The moulds also need to be long-lasting, be capable of rapid change of section sizes, and require the minimum of maintenance effort.
Continuous casting moulds are cooled by high cooling water, often demineralized, supplied from the recirculating system. The design and the fail-safe system are usually arranged to provide a minimum water flow velocity in the cooling channels of 8 m/sec. Moulds are invariably tapered internally to accommodate contraction of the steel but the amount of taper depends on the section sizes and the casting speeds involved.
The copper moulds are contained by steel backing plates with the water inlet and outlet manifolds at the bottom and top of the mould respectively. The water cooling grooves are machined at the back of the copper plates from top to bottom in slab and bloom moulds the dimensions of these being about 15mm deep and 5 mm wide. In billet moulds the water channel is usually a parallel gap between the tubular copper mould and the backing plate.
To ensure a thin boundary layer at the copper surface and hence no nucleate boiling, a high Reynolds number is required in these water cooling grooves which results in a need for the water velocities being greater than 8 m/sec.
A variety of cross-section shapes of different sizes can be continuously cast in accordance with the product requirements of the steel plant. Apart from the usual square, rectangular and round sections, hollow and polygonal section strands are also possible, as are performed blanks for subsequent beam production.
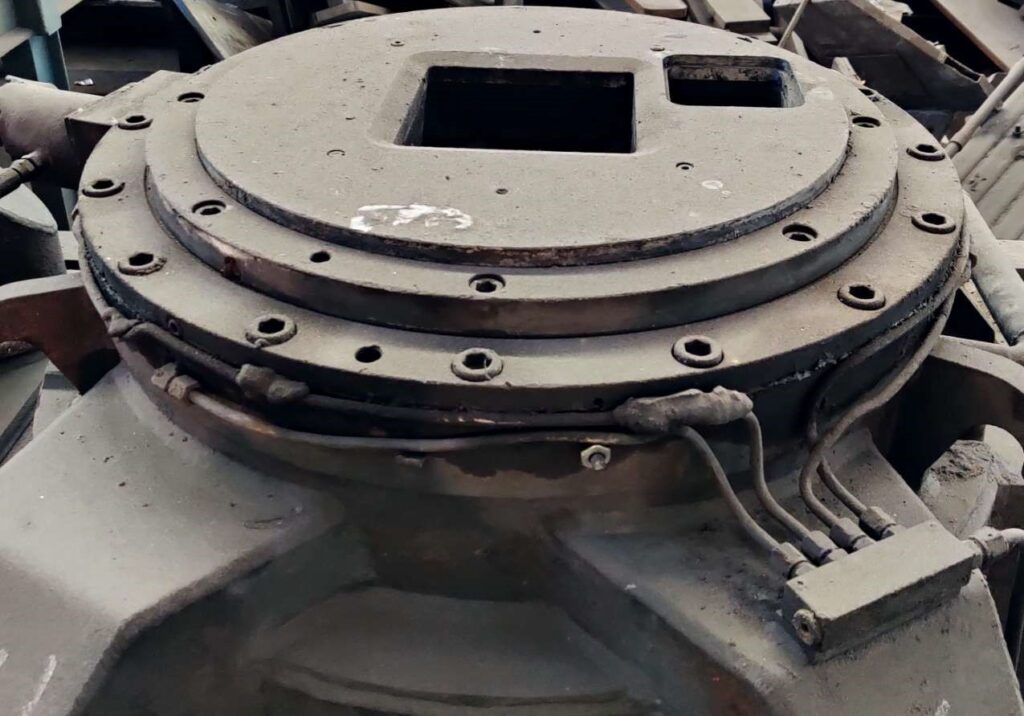
These different types of strand format naturally require different types of mould. Generally, moulds are classified according to three basic categories:
Block moulds (manufactured from a single forging)
Tabular moulds (manufactured from the seamless tube)
Plate moulds (Plate assembly)
All these moulds types, with the exceptions of moulds for hollow strand production, may be either straight or curved design.
The block mould, which is machined from a solid copper forging, is suitable for all strand shapes but does have two important disadvantages: firstly, its manufacturing costs are very high, and secondly reconditioning to its inner walls automatically means an increase in strand cross-section size.
Nowadays tabular moulds are manufactured from the seamless tubes and are employed for small square and rectangular shapes up to a side dimension of approx. 250 mm. For round strands, tabular moulds are employed right through the range to the largest diameters( currently 450 mm mould I.D).
For larger strand crosssections and particularly slabs, plate moulds are now used exclusively. These usually comprise two large copper plates for the broad faces with two narrow face plates located between them which can be moved in and out for width adjustment.
Design of the plate mould
The shaping walls of the moulds consist of rolled or forged plates in copper alloy. These are bolted to a sturdy steel box that acts as both support and cooling water jacket. The steel boxes (also called water boxes) are supported by a steel frame onto which the gearboxes for narrow face adjustment and the clamping mechanism are also mounted.
In curved moulds, the outer arc is the fixed side, it can, however, be adjusted to a small extent by means of an adjustment screw. The inner arc (floating side) can be hydraulically released for thickness adjustment. The narrow faces are power-adjustable for width changes.
Mould plates:
Broad faces: The general practise nowadays is to provide the copper plates with water channels at the back and to bolt them directly onto the water boxes. Fixing bolts evenly distributed over the plate area is supplemented by additional bolts to secure the plates outside the water box in order to avoid plate distortion at and above the meniscus level. Wide channels require water displacement plates in order to achieve a sufficiently high velocity of water flow. Small channels are more difficult to manufacture. Channels more than 90 mm wide ensure sufficient distortion resistance in the copper lining.
Mould cooling efficiency remains the same whether the cooling water flows through the mould jacket from top to bottom or from bottom to top. The main criterion is that the coolant flow does not break down, as interruption leads to vapour bubbles and thus uneven cooling and local overheating. Continuity of coolant flow is ensured, provided that the flow velocity is maintained above 3m/sec. In the case of machines with high casting speeds, water velocities of 10m/sec is recommended.
The depth of the cooling water channels roughly corresponds to the thread depth of the bolts (approx. 25mm). New plates should be approx. 60 mm thick where repeated re-machining is envisaged.
Design of the narrow faces
The copper plates for the narrow faces of the mould are of similar design to those used for the broad faces. However, they are usually somewhat thicker as experience has shown that they undergo a higher degree of wear. In width adjustable slab moulds, the water boxes with these copper liners are mounted on spindles driven by adjustment drives which can also be used to modify the narrow face taper. The broad face taper is achieved by employing narrow face plates of tapered design i.e. narrower at the mould outlet and wider at the mould inlet.
Water boxes and mould frame
The steel water boxes perform a dual function- they provide stable support for the bolted on copper liners and also ensure even distribution of the mould cooling water over the cooling surfaces.
A vent valve is provided at the highest point of the mould coolant circuit to allow the release of air pockets which may be developed in the piping as a result for example, of a mould change. The four water boxes in a plate mould are held together in a mould frame. This frame supports the necessary securing and adjustment elements and guides the various water circuit from and to water quick –connection plate on the oscillating table.
The mould frame is designed to facilitate mould and foot roll alignment on an alignment stand outside the casting machine, and also for fast, precise installation of the mould in the caster.
Mould foot rolls
Many casters feature foot rolls for supporting and guiding the thin strand shell at the mould outlet. The foot rolls, which oscillates with the mould, are adjusted so that the strand is guided centrally. This ensures that the strand shell is not subjected to any long bias and also that it is not pushed too heavily against one of the mould walls. Such conditions could lead to uneven cooling and thus to longitudinal cracking in the strand, strand deformation, excessive mould wear and possibly copper pickup by the strand leading to hot shortness.
Central strand guidance is equally important for both straight and curved moulds. The only difference lies in the type of alignment measuring devices required (straight edge versus arc template).
The foot rolls, like the following rolls in the guide segments, are subjected to heavy thermal loading which can lead to the rolls becoming bent. The foot rolls should be adjusted precisely to the dimensions of the mould outlet in order to maximize guidance efficiency.
Strand guidance immediately below the mould
Instead of foot rolls which oscillate within the moulds, many casters feature other guide and support elements.
Cooling plates of appropriate design can be an effective means of strand support. Their length varies from 0.5 and 1.5 m. The advantage of improved shell containment is however offset by the great disadvantage of excessive friction as the strand is withdrawn. Heat dissipation per unit area of the cooling plate is much less than the average heat value transferred from the mould to the cooling water. In order to improve the cooling effect, water may be introduced between the strand and the cooling plate. However, this practice is not recommended because, if a strand breakout should occur between the mould and the cooling plate, an explosion could occur.
Cooling grids cause less strand friction. Water is sprayed onto the strand surface through the openings in the grid in order to counter the disadvantage of a lower cooling rate. However, casting flux, scale and packing material which accompany the strand as it is withdrawn, often deposit in the openings, hindering uniform cooling.
Walking beam produces the lowest level of friction. The beams of appox 200 mm in width move down in contact with the strand then lift off again for the upstroke, producing a mild strand cooling effect. They are only employed for slab casting.
Mould length
Mould length varies between 500 and 900 mm depending on the production requirements of the individual company. The mould length is determined as a function of the casting speed. Heat transfer measurements show that high casting speeds require a longer mould. However, it has been observed that the mould length rarely exceeds 1000mm.
Mould plate material
The choice of mould material is crucial and it has to meet wide range of requirements determined by the casting process. Apart from having sufficient hardness in order to resist wear and mechanical damage, it must also exhibit a high level of thermal conductivity.
A number of different mould materials that have been tested in the past are copper, copper alloys, molybdenum, graphite, steel, and cast iron. The most successful of these in terms of economic and technical expediency have been copper and certain copper alloys. Graphite is too soft, whilst molybdenum is too brittle. Low carbon steel quickly results in plate distortion and deep fire cracks, caused by the high surface temperature which occurs during casting because of the material’s relatively low thermal conductivity. Similar problems are encountered with cast iron.
Copper and copper alloys
DHP copper
Unalloyed copper has a very low softening point which ranges between 140 and 180 deg centrigrade, depending on its purity. The softening point is taken as that temperature at which the material loses half the hardness induced by cold forming. DHP copper also undergoes continual shrinkage in high-temperature service owing to its poor creep characteristics. This disadvantage is particularly pronounced in the case of larger mould plates.
The thermal expansion which occurs during casting e.g 3.5 mm in the case of a plate width of 2400 mm results in plastic deformation and subsequent shrinkage when the copper plate cools down again. In the case of plates of hot rolled DHP copper, the shrinkage increases with the number of heat cast. Although the technologically significant properties of DHP copper can be improved by cold forming, they still remain unsatisfactorily for wide side moulds.
Alloyed Copper
The characteristics of the pure copper can be improved by the addition of various alloys and subsequent cold forming. The hardness, wear-resistance and creep behaviour of the copper all can be enhanced, by adding Cr and Zr. Addition of Co and Be can produce almost three times the Brinell hardness of DHP copper. However, this very hardness leads to premature mould plate failure due to cracking.
Silver alloyed copper (CuAg 0.1% P)
Among the copper alloys cold-formed, silver alloyed copper with a silver content of 0.1 % has become widely accepted as the material which is most suitable for slab mould plates. The main reason for this is its low tendency towards shrinkage and creep. The softening point lies in the region of approx. 320 deg C. The degree of cold deformation should be 20%. The thermal conductivity of the material is hardly affected by the low AG addition. The higher material costs are more than offset by lower repair cost and longer service life.
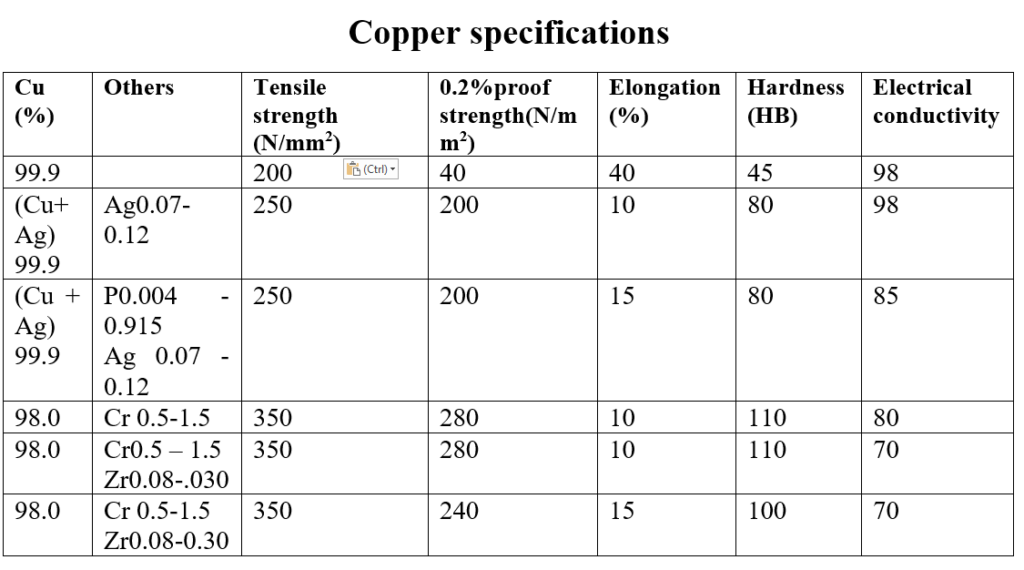
Coating of the mould internal surface
The wear resistance of these copper materials is low. Considerable wear occurs at the lower end of the mould in particular, and this results in a corresponding change in shape.
Electroplating the working surface with chromium in the hardness range HV 800-1000 usually involves a coating thickness of no more than 0.06 to 0.09 mm. Thicker coatings are only rarely applied. Owing to the soft Cu base, the hard Cr layer offers little resistance to mechanical damage. Tubular moulds, in particular, should preferably be provided with a hard chromium coating. Nickel coatings of approx. 4mm in thickness, applied by the explosive cladding method, start to develop fire cracks at the meniscus level after approx.. 150 heats and also begin to wear as a result of abrasion at the mould exit end.
Moulds can also be provided with laminated coatings comprising three layers in the form of
0.9 – 1 mm Nickel(electroplated)
30 – 50 µm Nickel-Phosphorus(chemically applied)
10 – 20 µm Cr (electroplated)
Thick Ni layers(1-3mm) are employed to increase the service life of the Cu plate to up to 4000 heats. With early galvanic nickel removal and recoating having to take place before the copper plate undergoes any wear, even higher lifetimes are theoretically possible.
Tests have been carried out involving the, for example the embedding of metal carbides in the Ni layer for increased wear resistance. The thickness of the Ni layers has also been varied(eg-3.0 mm at the meniscus level, 1-1.5 mm at the mould exit end) in order to improve the surface of the strand and reduce the depth of the oscillation marks.
Coating the copper plates with protective metals is not always required. It should only be carried out if rendered necessary by special conditions, eg. The occurrence of hot shortness. It may, for example, be sufficient to coat just the narrow faces of a slab mould if wear can be kept within limits by perfecting the operation of the oscillating table and ensuring the precise alignment of the foot rolls.
Mould taper
The strand which begins to for in the mould shrinks as it solidifies and cools. As a result the strand tends to come away from the copper wall, thus reducing at least partially – the support and guidance afforded by the mould.
The degree of shrinkage in absolute terms depends on the level of heat transfer from the liquid steel to the mould cooling water, the strand crosssection, the composition of the steel and the casting speed. In the mould, the strand shell may, after detaching itself from the mould wall, undergo a temperature rise which brings it back into the intimate contact. In slab moulds, the broad face of the strand shell is held permanently against the mould wall by the ferrostatic pressure acting upon it. Nevertheless, aslight downward taper of the broad faces of approx. 0.5% of the strand thickness can be of advantage.
The narrow face taper of a slab mould is given in % of the nominal width:
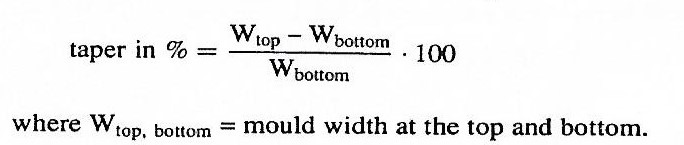
In practise, values between 0.6 and 1 % have proven most consistently successful for the &00 mm long standard mould. This range is the result of various parameters such as the steel analysis, casting temperature, casting speed and the method of lubrication. The lower values are usually applied where the casting speed is high.
In case of moulds for billets or blooms, the taper values are usually between 0.9 and 1 %. However, moulds with progressive reduced tapers are also used here because the largest degree of heat transfer, and thus the largest degree of shrinkage, occur in the first 100 mm below the meniscus.
Width adjustments during the casting process
In order to increase the availability of the continuous casters, particularly for the production of wide slabs as the starting material for the cold-rolled strip, the narrow faces of the slab mould are power adjusted to the required width during the casting process while the strand is still in the mould. This operation is either carried out so quickly that the new strand can be cast onto the previous strand while the latter is stationary, or performed without interrupting withdrawal so that the strand is given a tapered junction.
Without adjustment during stand standstill
Width adjustment during strand standstill is particularly suitable for the large width changes. Width differentials of 1000mm can be implemented. A stepwise adjustable mould is used for such an application in which the narrow faces are divided in half with a top and bottom section. When width adjustment is to take place, the metal level in the mould is allowed to fall to the bottom half of the mould, the casting operation is interuppted and the top halves of the narrow faces are repositioned for a narrower or wider strand. Then the bottom narrow face halves are also adjusted to the new width; if a narrower strand size is required, this second operation can only take place once the wider tail section of the previous strand has been completely withdrawn from the mould. The advantage of this system is that the change in width is abrupt (although several stages may be required for very large width changes), while its disadvantages are that the process requires an interruption to casting operations. The transverse division of the narrow face plates has also been known to cause problems.
Continuous Width adjustments
In the continuously width adjustable moulds, single-piece narrow faces are power adjusted inwards or outwards during the casting process. This imparts a tapered transition to slab strand which can be subsequently removed, e.g by torch cutting.
If the continuous system is combined with continuous tapered measuring device (e.g inclinometer) to monitor the narrow face taper, the mould taper can be continuously adapted to the prevailing casting conditions. In practical width adjustment operations, full consideration must be given to the various physical processes which occur as the strand becomes narrower or wider. If the narrow faces of the mould are adjusted inwards, they press against the narrow faces of the slab and produce a slight degree of deformation. The contact between the strand surface and the cooling surface of the mould remains intact and is even slightly improved. On the other hand, if the narrow faces are moved outwards, the contact between the mould and the mould wall is reduced. While the narrow faces are moving outwards the strand continues to shrink, producing an air gap. Thus the heat of the strand only is transferred at and just below the meniscus. Thereafter, the strand shell undergoes reheating and bulges. If narrow face adjustments is carried out simultaneously with the adaptation of the mould taper to the prevailing casting conditions, the speed of adjustment may be in the region of 200 mm/min. At a casting speed of 1.25 m/min this corresponds to a width change of 500 mm per 1m in slab length. It is reported that width adjustment during the casting process results in an increase in the production of 30-50%, a reduction in refractory costs by 30-50%, an increase in the yield of 0.3 -0.5%, and savings in energy of 30-50%.
Moulds for Twin and Triple Casting
Wide slab slitting is another method of achieved narrow slabs or blooms from the slab machine. The mould and top zone for twin or triple casting on a slab machine is basically two (or three) separate moulds and top zones, with two (or three) SENS for one “slab” strand on the tundish. The remainder of the strand remains the same so that the two (or three) blooms have a common withdrawal system. In some cases the wide slab mould with a central water copper divider is used rather than two separate bloom moulds. For a very large slab mould two slabs can be cast with widths up to around 1000 mm.